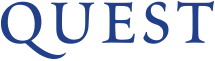
From Marconi's first transatlantic wireless transmission through Sir Henry Tizard's radar to modern cellular communications, the rapid advance of applied electromagnetics during the 20th century has changed our world. Now, in the 21st century, a new revolution in exploiting electromagnetism (EM) is emerging that brings together two recent developments - spatial transformations and the design and fabrication of novel electromagnetic materials. The idea of spatial transformations (STs) is to provide entirely fresh solutions to the distribution of the spatial arrangement of materials so as to enable new ways to manipulate the emission, propagation and absorption of EM radiation. This goes far beyond what can be accomplished with traditional materials in the form of lenses and mirrors, requiring both conventional materials and also those with properties that do not exist in nature (i.e., metamaterials).
To make the required exotic materials in large quantities, modern fabrication techniques will be needed, including the use of nano-composites and graded-index coatings. The material palate can be further widened by the inclusion of “active metamaterials” and superconducting dielectric composites.
The fundamental understanding of how EM radiation interacts with matter, and an exploration of how it can be controlled, is central to this project. Specifically, our interests lie in how an EM field within a material, or incident upon its surface, can be manipulated in order to achieve a desired response. On a macroscopic level, the elementary concepts of permittivity (ε) and permeability (μ) describe how the electric and magnetic fields behave locally. This knowledge in turn allows one to model how an EM wave may be controlled.
In the Consortium, we have world-leading expertise in the design and fabrication of novel EM materials, bringing together disciplines such as the physics of solids, micro- and nanofabrication, electromagnetic theory and computational methods, optics and microwaves, chemistry and nanoscience. This multidisciplinary approach will unlock the full power of electromagnetics, and make the practical application of Spatial Transformations a realisable target.
The power of the concept of Spatial Transformation is that it may be used to specify the required electromagnetic properties of a material in order to control in a predefined manner the path of an electromagnetic beam. These specifications typically require anisotropic and exotic material parameters that can only be achieved by the fabrication of novel materials, but lead to novel ways to control “electromagnetic space”.
Many interesting potential applications derived from different ST approaches have been proposed, and confirmed by simulations. In addition to the well-publicised cloaking phenomenon, these include all-angle absorbers and retro-reflectors, reflection-less beam shifters and beam-splitters, and “flat” parabolic mirrors. The fundamental ideas underpinning this project are already reasonably well established, and have been demonstrated in-silico for a limited set of cases. A major aspect of our programme is to establish a much wider toolbox of ST-based modelling codes to allow for a much more far-reaching exploitation of these evolving concepts.
While conventional, isotropic, “positive” index materials and composites provide the necessary electromagnetic response for a subset of STs (e.g., those involving graded-index media, such as Maxwell’s fish eye lens), we are extending our palette to include non-isotropic, multi-lithic materials, with effective material parameters (ε, μ) that simply do not exist in nature, and in which the EM properties vary in at least one dimension in a controlled and pre-designed way. There have recently been many new developments in material fabrication techniques for ‘designer materials’, including inkjet printing, selective laser sintering, etc., but these processes offer neither the scale nor the speed to fabricate the more ambitious demonstrators we envisage. New approaches to large area prototype manufacturing are under development.
There are a significant number of major challenges involved with developing QUEST devices, ranging from developing a modelling toolkit based on new spatial transformation to the innovation of practical manufacturing methods to fabricate novel EM materials and a set of demonstrator devices meeting the needs for our industrial partners.
The first obvious challenge is that not all EM problems that need a solution may be amenable to STs. One of the key tasks thus facing the theoretical development is to discover the bounds which may limit the ST toolkit. Secondly, while some problems will require one type of transformational approach, others may require quite different ones. The end users of the tools developed in this project will need to know which to use and will not want to have a special program for each specific situation. Defining the different types of generic practical situations and selecting which specific transformational approach is required to deal with them will be addressed in a robust way by the QUEST theoretical team.
On the materials side, the most obvious challenge when trying to provide as wide a range of effective values of permeability and permittivity as possible is that, for negative index materials, we must achieve magnetic resonance. This will inevitably be narrow band and will have associated loss. While for some specific applications, narrow band may be acceptable, in general loss is not. Thus, there will be a need to address the whole issue of loss compensation, including consideration of the inclusion of active compensation. Finding suitable active techniques for frequencies above 1 GHz is a far from trivial task. The narrow bandwidth will be a major problem for other applications and, as well as using the metamaterials route to try to meet this requirement (a formidable challenge), ways to avoid the need for negative index over a wide spectral band will be sought by the ST theorists. A further challenge is how to develop suitably anisotropic metamaterials, which will give the theoretical modellers a much more extensive range of transformation options, allowing them to explore ST beyond the locally isotropic domain. Of course, for layered structures with simple cylindrical or spherical symmetry, this may be not such a problem, since fabrication techniques for ‘Swiss roll’ type structures are reasonably well established, and these kinds of devices are the initial focus, with other designs considered as the project progresses.
On the device fabrication side, many demonstrator structures will require much more complex forms which will certainly not have such high symmetry. The need to arrange small (sub-wavelength) elements with local ‘designer’ permittivity and permeability values in complex geometries will be a major challenge. While laborious fabrication by a ‘Lego’ type approach may be acceptable for a one-off prototype, this will not be satisfactory if many equivalent structures are to be built and tested. Thus, the project is faced with the major challenge of how to produce arrays of sub-wavelength elements which are easy to assemble, or inherently self-assemble, into a wide range of 2D and 3D structures.
Our programme of study is spearheaded by three distinct but interlinked projects underpinning the science behind the innovation and design of QUEST devices. These are: the conceptualisation of novel spatial transformations (SP1); the establishment of a computational toolkit for the design of QUEST devices based on these concepts (SP2); and the practical development, manufacture and electromagnetic characterisation of novel materials for use in QUEST devices (SP3). The final SP (SP4) provides an experimental test bed for QUEST devices and is designed to deliver feedback to the first three SPs.
Flexibility is built into all the SPs to ensure that the Consortium can respond rapidly to developments in this competitive field as the programme proceeds.